Download this White Paper:
The increasing importance of limiting emissions to the atmosphere draws attention to the biggest culprit for this in conventional pumps, the mechanical seal. Emissions not only pose a safety and environmental risk, they also have a huge impact on maintenance costs and lost time of production.
Sealless pumps are such that they do not use a mechanical seal that leaks into the atmosphere, thus eliminating the possibility of leaking, dangerous or otherwise, fluids into the environment. This category of pumps offers additional simplicity in eliminating coupling alignment, bearing frame maintenance and auxiliary systems associated with lubricating or cooling bearings.
The two main categories of sealless pumps are canned motor pumps (CMPs) and magnetic drive (sometimes called mag drive). CMPs offer increased safety through double containment, a reduced number of bearings (and inherent maintenance costs) and improved reliability when applied properly to the application.
How is the Seal Eliminated?
Due to the laws of physics, higher-pressure fluids want to move to lower-pressure areas. Because traditional pumps must couple to a driver (motor or turbine), the shaft needs to exit the pump pressure boundary (pump case). This means the high-pressure fluid created by the rotating pump wants to leak into the atmosphere along the shaft penetration. This is stopped in traditional pumps by using gland packing or a mechanical seal.
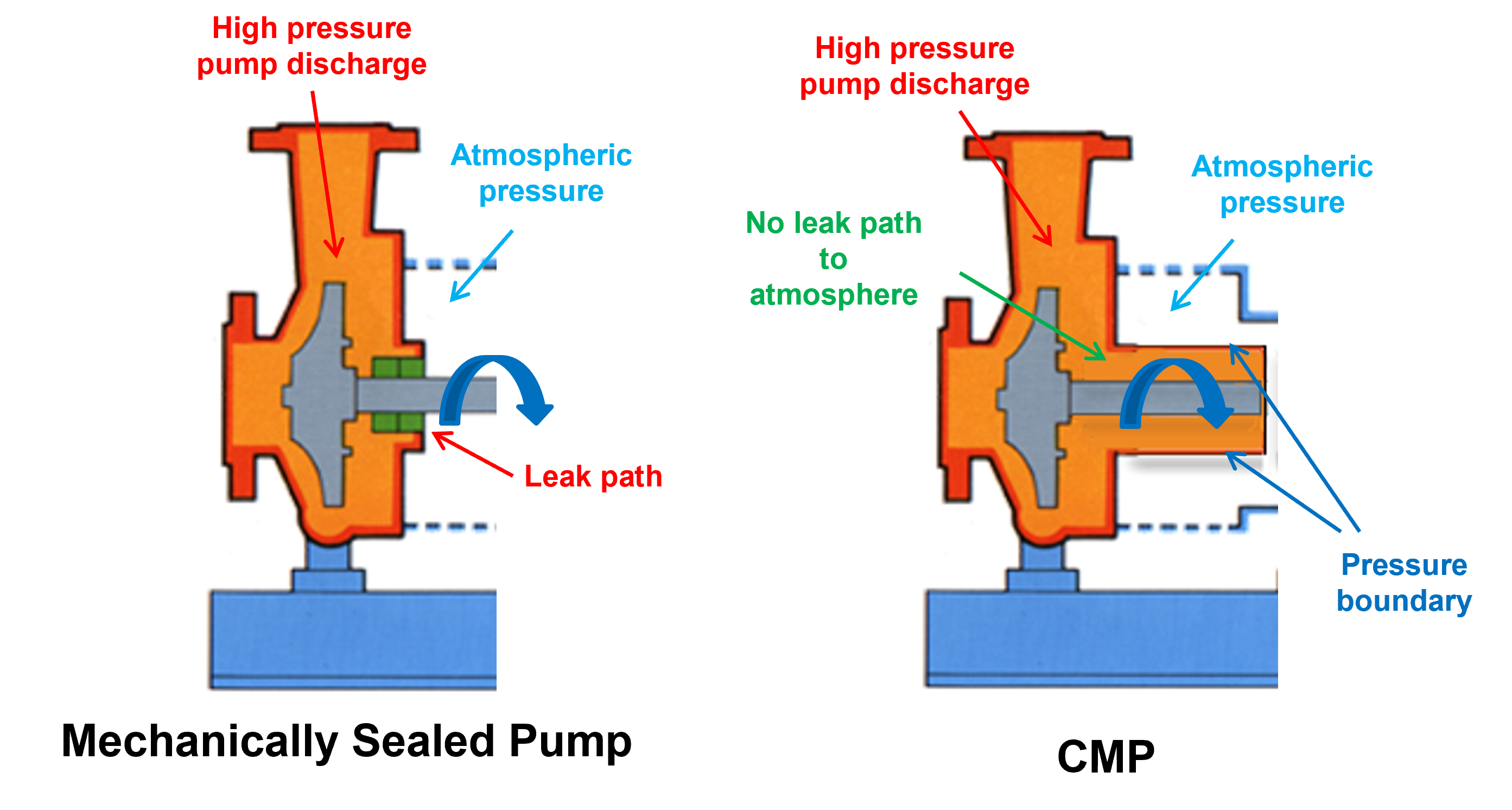
CMPs use a different approach by using a common shaft for the pump and motor and containing it all in a pressure boundary. This eliminates the pressure differential across the rotating seal and the driving force for the fluid to leak. Using a common pump and motor shaft has other benefits, such as eliminating coupling alignment (both hot and cold), therefore eliminating this potential failure point in the pump.
Materials Considerations
CMPs are constructed of materials compatible with most process fluids yet are not overly expensive. The typical metallurgy of wetted components of a canned motor pump are type 304 stainless steel with the stator and rotor cans made from Hastelloy C276. Although Hastelloy C, a nickel-based alloy, is more exotic and expensive compared to 300 series stainless steel, the higher electrical resistivity significantly reduces the eddy current losses in the stator can, and to a smaller degree, the rotor can. Overall, using Hastelloy results in increased efficiency of the motor.
Additionally, since the stator and rotor cans are fabricated from a thin material (0.010 inches to 0.015 inches) to minimize electrical losses and reactivity, it is important to use strong and highly corrosion-resistant materials. The cans are further supported by the electrical steel laminations along the length of the lamination pack and with heavier-duty backup sleeves at the wire coil end turn regions. These support the thin can in dealing with the high internal pressures (up to 6000 pounds per square inch [psi]/42 megapascal [MPa]) within the motor.
For sealing of joints, there are typically face-to-face gasket designs and O-ring gaskets with a machined groove. For both types of joints used to seal the pump-to-motor and motor-to-end cover mating surfaces, it is important to verify the compatibility of the material with the process fluid. A standard design uses polytetrafluoroethylene (PTFE) gaskets due to their vast compatibility with various process fluids. For higher temperature and pressure applications (above 390 F and 580 pounds per square gauge [psig]), a spiral wound stainless steel is typically used. The grade of stainless steel will be matched to the pump case. For special chemicals or specific applications, other gaskets may be used, and the individual compatibility of those fluids with the gaskets must be assessed.
If using an O-ring joint design, a Teflon encapsulated Viton O-ring is common, as they provide the corrosion resistance of Teflon and the elastomeric resilience of Viton.
The bearing material for CMPs generally falls between two materials: carbon graphite and silicon carbide. An important point to understand with CMPs is they use fluid film bearings.
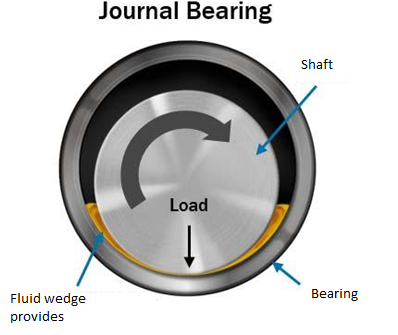
This means during operation the rotating surface of the shaft (or shaft sleeve) does not come in contact with the stationary bearing. Instead, as the shaft rotates, it creates a fluid film, and the fluid is forced between the shaft and the bearing. The typical design uses a hardened polished metallic surface on the rotor and softer corrosion-resistant bearing material. The bearing material is designed to be easily replaceable during periods of maintenance. Carbon graphite grades typically offer a softer, more forgiving bearing that is more tolerant of particulates and upset conditions but that will not last as long as silicon carbide grades. Conversely, silicon carbide being a harder material will last longer in the right application but is less tolerant of particulate wear and upset conditions. One material is not necessarily better than the other, and material selection should be based upon the application and user requirements/preferences. Other materials, such as polyether ether ketone (PEEK) and other composites, can also be used but are less common.
Consideration of Motor Fluid Properties
When considering the bearing lubricity and motor cooling, it is necessary to understand the requirements and characteristics of the canned motors as they relate to the fluid properties. Typically, the pumped fluid lubricates the bearings and cools the motor. In some instances where the pumped fluid is not compatible with the motor (too much particulate, viscosity issues, etc.), then a barrier fluid can be used in the motor.
The motor fluid must have appropriate viscosity to provide adequate lubrication of the hydrodynamic bearings yet have sufficient flow through the motor to cool, all while keeping fluid friction drag losses to a minimum. Generally, fluids with an absolute viscosity greater than 200 centipoise and less than 0.07 centipoise are not desired. An alternative solution when the pumped fluid cannot be used inside the motor involves a barrier fluid. A labyrinth seal is used to isolate the pump, and the motor is filled with a compatible fluid different than the pumped fluid. It should be noted in some cases an internal mechanical seal is used. This seal does not leak to the atmosphere, but instead leaks a small amount of motor fluid into the pump end. With either sealing option, it is essential to ensure the compatibility of the motor (barrier) fluid with what is being pumped.
As previously mentioned, the fluid inside the motor is used to cool the motor, along with lubricating the bearings. The fluid will therefore increase in temperature as it circulates through the motor. As the fluid temperature increases, so does the fluid vapor pressure (and typically the viscosity decreases). For these reasons, it is important to evaluate the thermodynamic properties of the motor fluid and ensure it will not vaporize or “flash” in the motor. This might cause vapor lock and/or insufficient bearing lubrication.
The temperature rise of the motor fluid is not only a function of the amount of waste heat generated by the motor’s inefficiency, but also of the specific gravity, specific heat (heat capacity) and the flow rate of the fluid through the motor. In addition, the thermal conductivity of the fluid will affect the heat transfer rate from the motor, and ultimately, the temperature rise of the motor windings. Motor winding wire has an insulation class determining the maximum temperature the wire can be exposed to. Typically, CMPs use Class H (180 C) or Class N (200 C), although other ratings are available. Note that class designations for those above Class H are typically specified in International Electrotechnical Commission (IEC) 60085.
Taking this into consideration, there are six main fluid properties of the motor fluid that are to be considered for a given application. These are:
- specific gravity
- specific heat
- viscosity
- vapor pressure
- freezing point
- thermal conductivity
As the viscosity and vapor pressure of some fluids can vary significantly with temperature, it is best practice to understand these properties at various temperatures. This would generally be at normal operating, minimum operating and maximum operating temperature. It is worth noting most manufacturers have a wealth of knowledge on various commonly pumped fluids, so they can offer support in defining these if they are unknown at the specification stage. As the viscosity and vapor pressure properties do not vary linearly with temperature, the three points mentioned can be used to generate an approximate curve providing these values to be calculated for other temperatures.
Motor Circulation Flow Paths
There are a variety of fluid flow paths for circulating fluid through the motor. Most manufacturers adopt those specified in the American Petroleum Institute (API) 685- Sealless Centrifugal Pumps for Petroleum, Petrochemical, and Gas Industry Process Service-Annex D. This standard outlines 14 different flow plans. Those familiar with mechanical seal plans will be accustomed to this concept. All these plans will not be reviewed in this article however the most used circulation plans are discussed. It is not an expectation that the user would identify the model type/circulation plan for their application, this would be done by the manufacturer’s Application Engineers. However, there are considerations that the user should know about when specifying a Canned Motor Pump, e.g. availability of cooling water (when pumping a hot liquid) or availability of pipework to route back to a tank (for low-vapor pressure fluids).
Plan 1-S Internal Circulation (Hollow Shaft)
This is the most common circulation plan and involves a small amount of the pump discharge circulating through orifices in the pump end (PE) bearing housing and into the motor. It travels through the pump end bearing, across the rotor, and then through the cover end (CE) bearing before returning to the suction via the hollow rotor shaft.
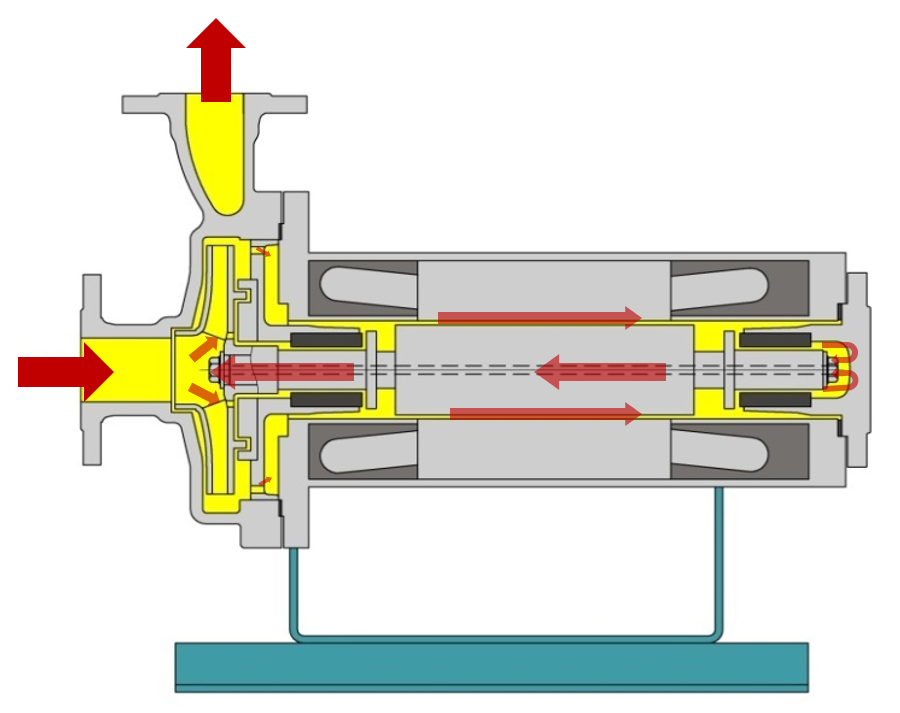
Plan 1-SD Pressurized Circulation
This circulation plan is used for handling volatile fluids that have a low boiling point. The premise is that the auxiliary impeller adds pressure to the motor cooling fluid to prevent it from vaporizing inside the motor. The flow path starts from the pump discharge and travels through ports to a hole in the hollow shaft. It travels through the hollow shaft to the eye of the auxiliary impeller. The flow splits and some goes through the CE bearing and back to the aux. impeller and the rest travels past the rotor, thought the PE bearings and back to the discharge side.
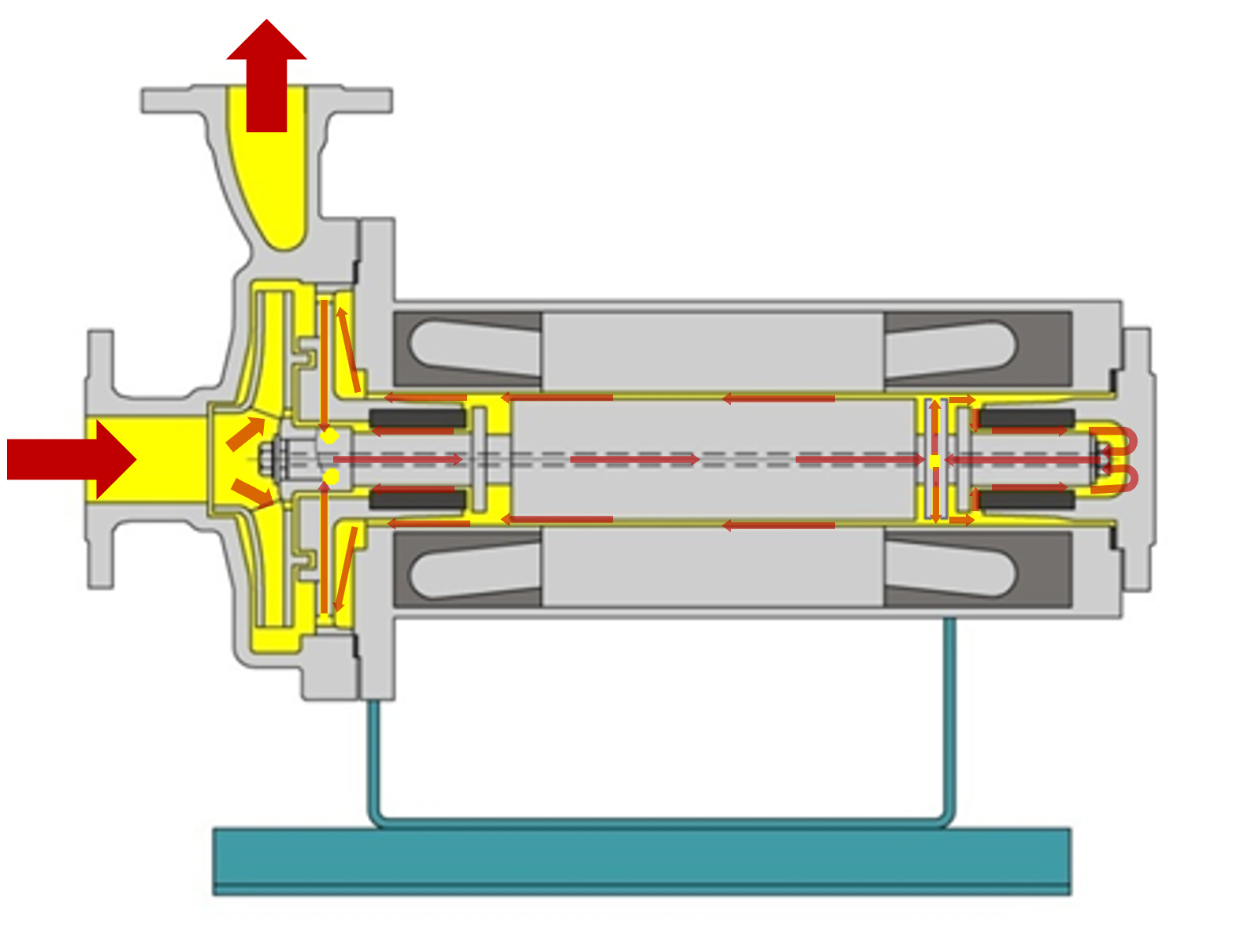
Plan 13-SE Reverse Circulation
This circulation plan is used when pumping fluids with low vapor pressure, e.g., refrigerants, liquefied gases, ammonia, etc. The fluid is circulated only once through the motor to avoid excessive heat pick up in the fluid and hence cavitation. As discussed previously, any vapor inside the canned motor pump can do significant damage to the bearings and other internal parts.
The flow path is from the pump discharge through holes in the PE bearing housing, through the PE bearing, across the rotor, and through the CE bearing before exiting the motor at the cover end. The fluid would typically be returned to a suction tank or collection manifold.
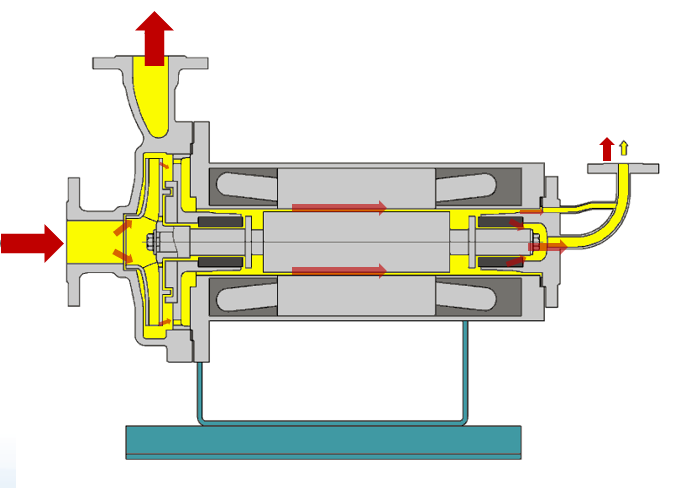
Plan 23-S Externally Cooled Motor
This circulation plan is used when pumping high-temperature liquids, for example, heat transfer oils. This circulation uses a heat exchanger to cool the motor fluid. The same fluid being pumped is used inside the motor, just at a lower temperature. The design uses a thermal barrier to thermally isolate the hot pump end from the cooler motor. This circulation requires site-supplied cooling water or can use an air heat exchanger, although this is less common. Generally, a small amount of fluid circulates from the impeller discharge, through the thermal barrier, and toward the heat exchanger process inlet. The motor fluid circulates through the heat exchanger at the PE and then enters the motor at the CE. It passes through the CE bearings, across the rotor, and through the PE bearings before repeating this circuit. There is sometimes an auxiliary impeller keyed to the shaft to help circulate the motor fluid which can be located at either of the bearing housing ends.
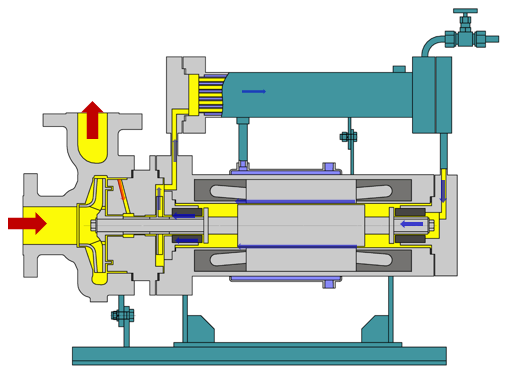
Plan 1-S High Temperature No Cooling
Although this uses the same circulation path as highlighted previously it is worth mentioning as an option for pumping high-temperature fluids when there is no site cooling available. This motor design uses an advanced grade of insulation (class 400) for handling higher temperatures. This motor configuration is typically only available up to 120 horsepower and is often not rewindable
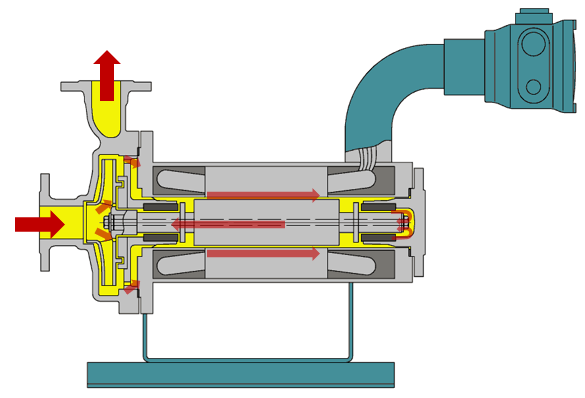
Plan 53-S and 54-S Slurry Handling
This circulation plan is suitable when pumping slurries or abrasive fluids that do not provide good lubrication and cooling to the motor. This plan uses a barrier fluid inside the motor. The barrier fluid is circulated through the motor either originating from a seal pot (53-S) or an external source (54-S). A labyrinth seal (or internal mechanical seal) separates the pump end from the motor. An external metering pump is commonly used to ensure the motor pressure stays above the pump discharge pressure. The compatibility of the motor fluid and the pumped fluid must be checked as a small amount will leak from the motor to the pump across the internal labyrinth seal/mechanical seal.
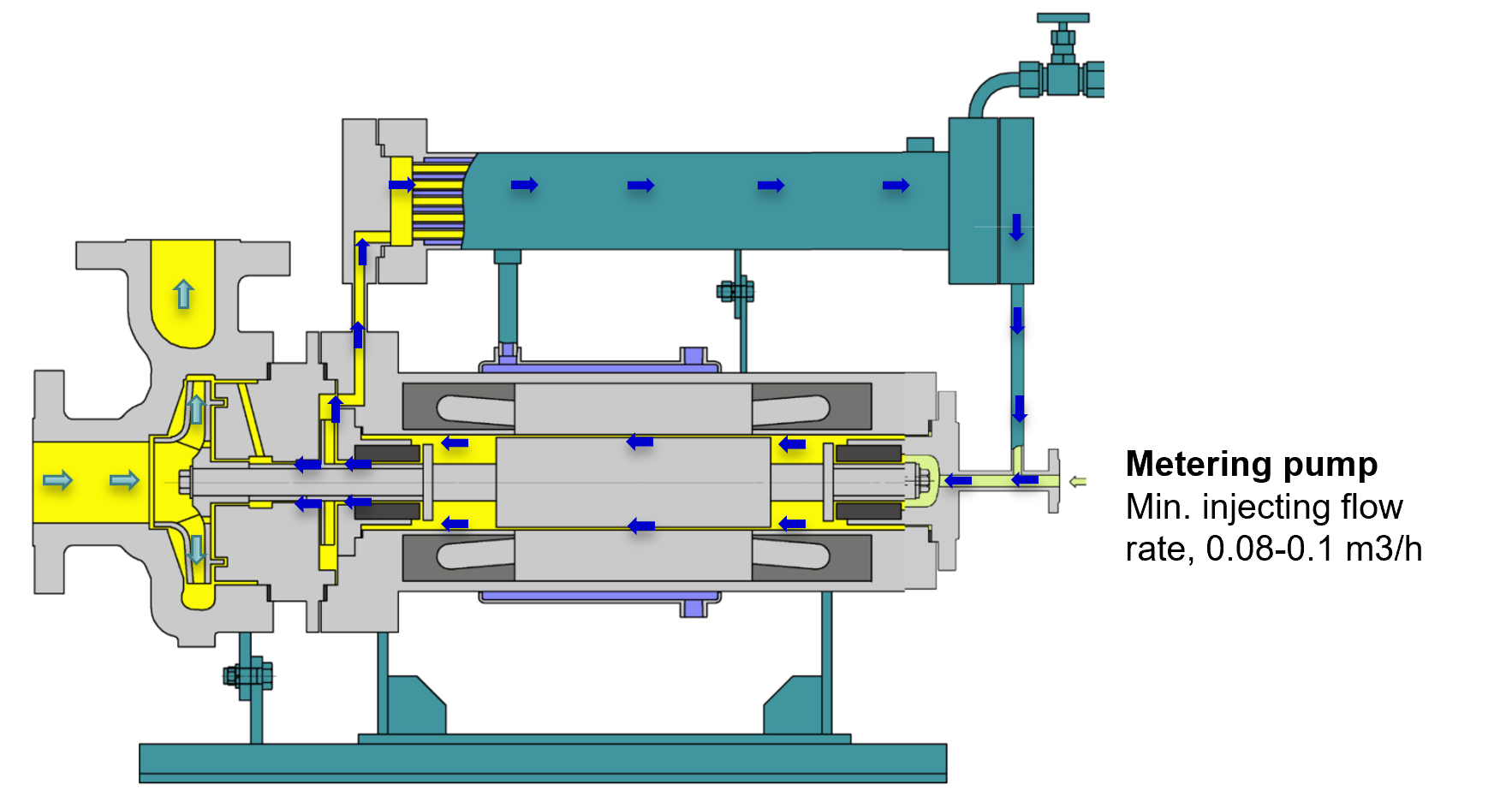
Conclusion
When investigating the use and benefits of a canned motor pump, whether a new installation or a retrofit of an existing pump, it is essential to know the fluid properties and operating conditions. Your analysis must include expected abnormal or possible upset conditions, which can affect its performance and reliability. This requires a mutual understanding of the application considerations by the system designer, end user, and the canned motor pump manufacturer. With this approach, a cost-effective and reliable installation will be realized.
Canned Motor Pumps offer the safest and most environmentally friendly pumping option when handling fluids that can harm the environment. Canned Motor Pump use is rising for these reasons combined with features that eliminate the most common failure modes for pumps. Eliminating the mechanical seal, removing the requirement for hot and cold alignment, and reducing the number of bearings plus lubrication systems offer a more reliable and maintenance-free pumping solution when correctly applied to the application.